Introducing Weria Pezeshkian
Weria Pezeshkian is a newly appointed Assistant Professor in the Niels Bohr International Academy where he joins a burgeoning community of biophysicists, who share an aim of pushing physics into biology across UCPH.
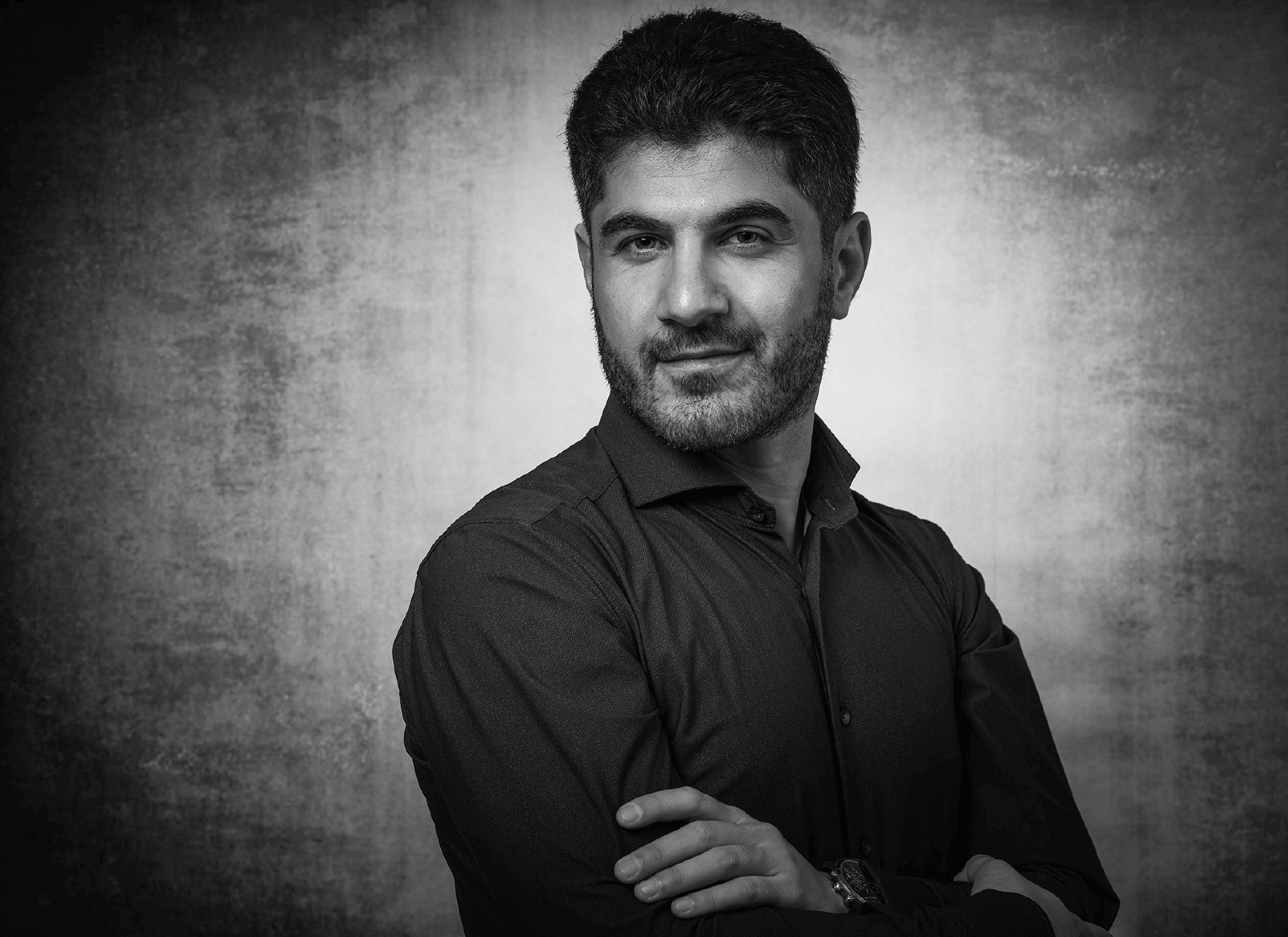
Can you tell us about the multi-scale approach that you have pioneered?
The multi-scale simulation is like a microscope. The reason we call it a microscope is that you can really access certain information by looking at it, like the dynamics of molecules, and their movements. You can zoom in and out. But it is a computational microscope, inside a computer.
Usually, protein modelling is done at an atomistic level and sometimes also at the coarse-grain level. What I have added is a meso-scale which is a bit larger. Each protein in the meso-scale is represented by one single particle and inside each particle is many equations to represent all the important information for this scale that we know from the atomistic and coarse-grained scales. But at the meso-scale we study how these proteins act collectively; not a single one but how they act all together.
A true thing with biology is that one plus one is not two. What I mean is that if you study one protein you don’t know what happens if you have one hundred or hundreds of them. There are new behaviors that appears that we often refer to as emergence. And this is what this computational microscope can show you.
What led you to develop the multi-scale simulation?
Membranes are my favorite biomolecular complexes and I study how they behave. During my PhD I got interested in how Shiga toxins enter the cell. You can give a vague interpretation of this phenomenon and say that these proteins (toxins) bind to the membrane, induce a curvature in the membrane, cluster, make a tube and then go into the cell. This is very descriptive, not wrong, but not very explanatory.
What is important is that what happened in the single protein? What does a single protein do to a membrane? To answer these questions, you have to look at the atomic level and say that this toxin actually binds to the membrane like this and induces the curvature like this. But then you want to see how they cluster. At the atomic scale you can’t obtain useful information by studying two Shiga toxin particles, you can only study one, so you have to go to the coarse-grained scale. And when you do this, you have to take all the important information from the atomic level, you can’t just construct a coarse-grained model. But then you want to find out what happens when there are 200 of them and for that, you have to go to the meso-scale. But again, you can’t just create 200 of them, you have to first have the data from the atomic and coarse-grained levels. The phenomena itself is multi-scale so the approach needs to be multi-scale.
And how did you apply this method to the study of Shiga toxins?
There is an effect in quantum physics called the Casimir effect. A vacuum has a fluctuation and if you put two conductor plates facing each other inside a vacuum, these plates will suppress the vacuum fluctuations. Because the vacuum wants to have more fluctuations, these plates attract each other. Using the multi-scale simulation, we have applied the same idea to membranes.
Membranes are not just static sheets, they are always undulating up and down and these are structured fluctuations, these are not random noise. When proteins tightly bind to the membrane, they perturb these fluctuations and the membrane will be unhappy about it and will push the proteins together and so they cluster. What we showed was that the Shiga toxin actually harnesses this force. Due to this suppression of fluctuations, suddenly they cluster and when they cluster, together they create a tube which they can then use to enter the cell.
We predicted this through simulation and our collaborators tested the predications, using synthetic chemistry, FCF experiments on unilamellar vesicles and showed that this is actually how it happens (. In the recent years, more theoretical and experimental data has supported our hypothesis.
How do you build a bridge between your computational approach and experimental approaches?
While I was doing my PhD at the Center for Biomembrane Physics, SDU, I was also a Marie-Curie fellow in which I was the only theoretical physicist in the consortium. The rest of the group were experimentalists, mostly cell biologists. I did my Masters in soft matter physics in Iran so it was a bit hard coming from a physics background and suddenly meeting so many biologists, you have to learn the language.
For example, physicists don’t like specificity. We usually say if you have a hydrophobic domain, this happens. But a chemist or a biologist really want to see which amino acid does that, or which structure does this and how sensitive it is. And physicists don’t like names. Biologists they have a lot of names for different parts of the systems whereas we just look at the general behaviors. It took me more than a year to learn the language.
Being in the Marie-Curie network really helped. I actually spent time at the Curie Institute in Paris to do some cell biology experiments to understand how biologists interpret their data. I did some cell culturing, some fluorescence microscopy and imaging. I saw how membrane nanotubes look like under a microscope. Mostly there was a Postdoc helping me a lot. That was a good fit.
How did you end up at the Niels Bohr International Academy?
I did my PhD in molecular biophysics at SDU in Odense. I then stayed there to do a Postdoc. After my postdoc in Odense I went to Marrink laboratory in Groningen. The group is called Molecular Dynamics Group - often referred to as Martini group, after the Martini force field, not the drink - and they were pioneers of this field. So I actually went there to couple the multi-scale simulation method to the technique that they were using and that was very successful. But I always liked Denmark. When I left to go to the Netherlands, I always planned to come back to Denmark. In general, I like the academic environment in Denmark, I like the culture as well, and I like the social system. There was an open position here at the Niels Bohr International Academy and I applied. Here it is mostly theoretical physics but now we are growing in a new direction, biophysics.
What opportunities are there for ISBUC researchers to collaborate with your lab
One of our aims is to push physics into biology and I am very interested in collaborating with experimental biologists. By combing the structural data with the simulation data, we can find out why such a structure exists.
I am less interested in single proteins but I am very interested in collective systems of a larger scale. I am especially interested in membrane related systems, lateral heterogeneity and other systems that have a membrane shape. Even viruses actually; we have done a simulation of the corona virus and resolved its structure using a multiscale simulation.
It would also be fantastic to find someone who can do experiments to test the models we make. Typically, it takes a long time to develop a new experiment so usually it is easier when computationalists collaborate with experimentalists on their projects or do computations for experimentalists. But I would be very happy to find someone to work the other way around, especially someone who does Cryo-ET because now we have a method where we can take Cryo-ET data directly and simulate organelle structures as we did with a small mitochondrion membranes. This is what we developed in Groningen.
Read more:
To find out more about my research on Shiga Toxins, see: Pezeshkian W, Shillcock JC, Ipsen JH. Computational Approaches to Explore Bacterial Toxin Entry into the Host Cell. Toxins (Basel). 2021 Jun 28;13(7):449.
and: Pezeshkian W, Gao H, Arumugam S, Becken U, Bassereau P, Florent JC, Ipsen JH, Johannes L, Shillcock JC. Mechanism of Shiga Toxin Clustering on Membranes. ACS Nano. 2017 Jan 24;11(1):314-324.
To find out more about the multi-scale method and simulation of membrane shapes: Pezeshkian W, Marrink SJ. Simulating realistic membrane shapes. Curr Opin Cell Biol. 2021 Aug;71:103-111.
To find out more about coupling the multi-scale method to Cryo-ET data, see: Pezeshkian, W., König, M., Wassenaar, T.A. et al. Backmapping triangulated surfaces to coarse-grained membrane models. Nat Commun 11, 2296 (2020).